
rexresearch.com
Rahul NAIR, et al.
Graphene Oxide Desalination
http://www.ibtimes.co.uk/graphene-sieve-turns-seawater-into-clean-drinking-water-technology-can-be-scaled-1615113
Graphene sieve turns
seawater into clean drinking water – and the technology can
be scaled up
Filtration system separates salt from
seawater, raising hope for millions of people who lack clean
water.
By Hannah Osborne
Graphene has been used to turn seawater into clean drinking water.
The graphene sieve was shown to filter common salts from the
water, and scientist say the technology could be scaled up –
potentially providing hope to the millions of people around the
globe who have limited access to clean water.
Graphene – often dubbed a wonder material – was first isolated by
scientists in 2004. It is just one atom thick, extremely light and
around 200 times stronger than steel. It is highly flexible and an
excellent conductor of heat and electricity, making it of huge
interest to scientists in real-world applications.
Scientists at the University of Manchester have now shown how
graphene-oxide membranes can be used as an efficient filtration
system. Publishing their findings in the journal Nature
Nanotechnology, the researchers were building on previous work
showing how these membranes could be used to filter nanoparticles,
organic molecules and salts.
The team had shown how when immersed in water, the graphene-oxide
membranes would swell up, causing larger salt ions or molecules to
be blocked out. Building on this, they created a sieve that stops
the graphene membrane from swelling when exposed to water, meaning
the pore size can be controlled. As a result, they could filter
out common salts from water (like seawater), making it safe to
drink.
"Building on these findings, we demonstrate a simple scalable
method to obtain graphene-based membranes with limited swelling,
which exhibit 97% rejection for NaCl [sodium chloride]," they
wrote.
The discovery has huge real-world implications. An estimated 663
million people worldwide do not have access to clean water close
to their homes, with many having to travel long distances or queue
for hours to get it. Under its current Sustainable Development
Goals, the United Nations hopes to make sure everyone on the
planet has access to safe water by 2030.
But this will not be easy. Climate change is putting more people's
water security at risk – increased droughts, flooding and melting
glaciers all lead to greater risk of shortages. And this, in turn,
threatens food production, sanitation, energy and industry.
graphene
The researchers said their graphene sieve can be scale up for
industry, and membranes can be created with "on-demand
filtration", meaning ions can be removed according to their sizes.
Corresponding author Rahul Nair said: "Realisation of scalable
membranes with uniform pore size down to atomic scale is a
significant step forward and will open new possibilities for
improving the efficiency of desalination technology.
"This is the first clear-cut experiment in this regime. We also
demonstrate that there are realistic possibilities to scale up the
described approach and mass-produce graphene-based membranes with
required sieve sizes."
Researchers hope the technology could be built on scales that
allow it to be used by countries without the financial
infrastructure to create large plants for water filtration.
In a related News & Views article, Ram Devanathan, from the
Energy and Environment Directorate at the Pacific Northwest
National Laboratory, said the sieve was a "promising approach to
treat industrial wastewater".
He added, however, that it will be some time before the sieve is
commercially viable. "The ultimate goal is to create a filtration
device that will produce potable water from seawater or wastewater
with minimal energy input," he wrote. "Much more work remains to
be done to produce graphene-oxide [GO] membranes inexpensively at
an industrial scale, ensure the stability and durability of the GO
laminate under prolonged operation with seawater, and enhance the
resistance of the membrane to fouling by organics, salt and
biological material."
https://www.nature.com/articles/nnano.2017.21.epdf
Nature Nanotechnology
Tunable sieving of ions using graphene oxide membranes
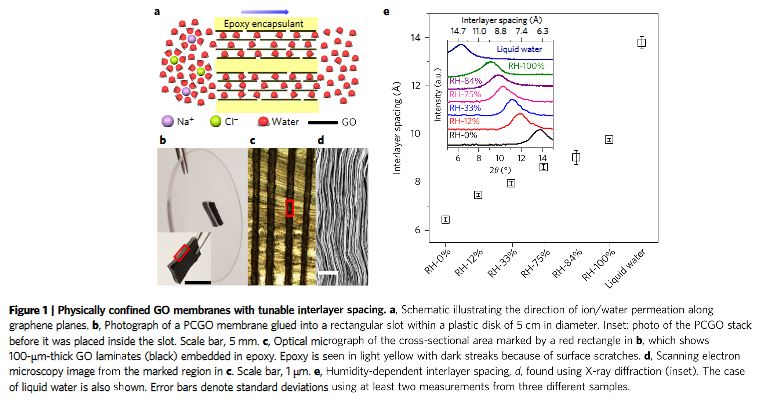
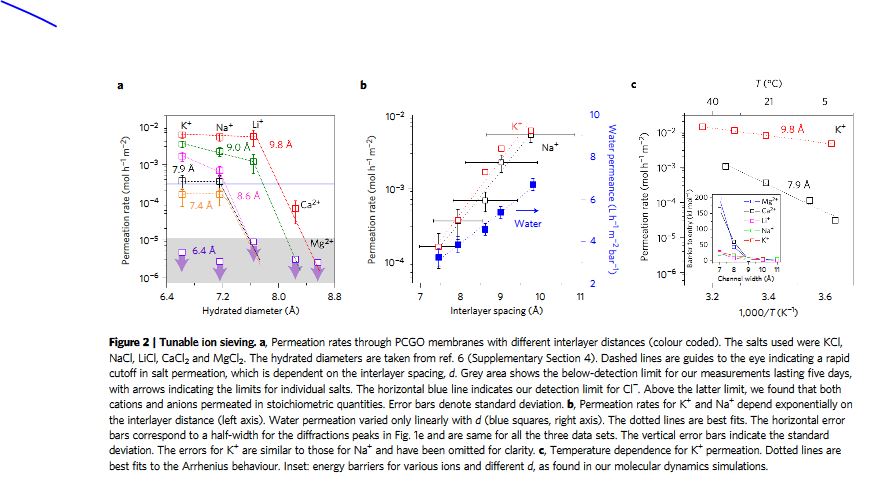
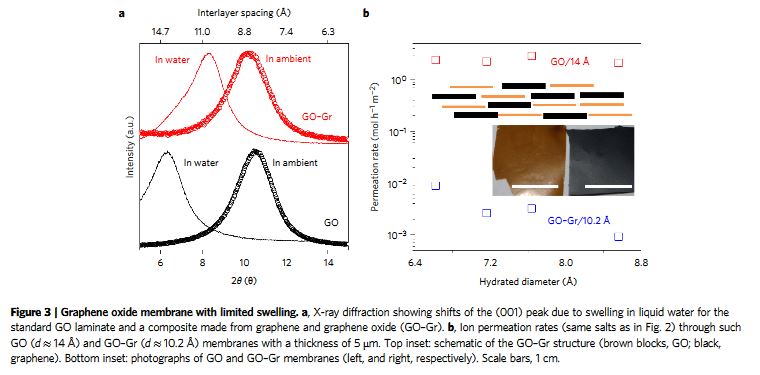
WO2016189320
WATER PURIFICATION
Inventor(s): NAIR RAHUL RAVEENDRAN, et al.
This invention relates to methods of purifying water using
graphene oxide laminates which are formed from stacks of
cross-linked individual graphene oxide flakes. The laminates also
comprise graphene and/or at least one cross-linking agent. The
invention also relates to the laminate membranes themselves.
O does not accept any responsibility for the accuracy of data and
information originating from other authorities than the EPO; in
particular, the EPO does not guarantee that they are complete,
up-to-date or fit for specific purposes.
Water Purification
[0001] This invention relates to methods of purifying water using
graphene oxide laminates which are formed from stacks of
cross-linked individual graphene oxide flakes which may be
predominantly monolayer thick. The laminates also comprise
graphene and/or at least one cross-linking agent. The invention
also relates to the laminate membranes themselves.
BACKGROUND
[0002] The removal of solutes from water finds application in many
fields.
[0003] This may take the form of the purification of water for
drinking or for watering crops or it may take the form of the
purification of waste waters from industry to prevent
environmental damage. Examples of applications for water
purification include: the removal of salt from sea water for
drinking water or for use in industry; the purification of
brackish water; the removal of radioactive ions from water which
has been involved in nuclear enrichment, nuclear power generation
or nuclear clean-up (e.g. that involved in the decommissioning of
former nuclear power stations or following nuclear incidents); the
removal of environmentally hazardous substances (e.g. halogenated
organic compounds, heavy metals, chlorates and perchlorates) from
industrial waste waters before they enter the water system; and
the removal of biological pathogens (e.g. viruses, bacteria,
parasites, etc) from contaminated or suspect drinking water.
[0004] In many industrial contexts (e.g. the nuclear industry) it
is often desirable to separate dangerous or otherwise undesired
solutes from valuable (e.g. rare metals) solutes in industrial
waste waters in order that the valuable solutes can be recovered
and reused or sold.
[0005] Graphene is believed to be impermeable to all gases and
liquids. Membranes made from graphene oxide are impermeable to
most liquids, vapours and gases, including helium. However, an
academic study has shown that, surprisingly, graphene oxide
membranes having a thickness around 1 μηι which are effectively
composed of graphene oxide are permeable to water even though they
are impermeable to helium. These graphene oxide sheets allow
unimpeaded permeation of water (10<10>times faster than He)
(Nair et al. Science, 2012, 335, 442-444). Such GO laminates are
particularly attractive as potential filtration or separation
media because they are easy to fabricate, mechanically robust and
offer no principal obstacles towards industrial scale production.
[0006] Sun et al (Selective Ion Penetration of Graphene Oxide
Membranes; ACS Nano 7, 428 (2013)) describes the selective ion
penetration of graphene oxide membranes in which the graphene
oxide is formed by oxidation of wormlike graphite. The membranes
are freestanding in the sense that they are not associated with a
support material. The resultant graphene oxide contains more
oxygen functional groups than graphene oxide prepared from natural
graphite and laminates formed from this material have a wrinkled
surface topography. Such membranes differ from those of the
present invention because they do not show fast ion permeation of
small ions and also demonstrate a selectivity which is
substantially related to chemical and electrostatic interactions
rather than size of ions.
[0007] This study found that sodium salts permeated quickly
through GO membranes, whereas heavy metal salts permeated much
more slowly. Copper sulphate and organic contaminants, such as
rhodamine B are blocked entirely because of their strong
interactions with GO membranes. According to this study, ionic or
molecular permeation through GO is mainly controlled by the
interaction between ions or molecules with the functional groups
present in the GO sheets. The authors comment that the selectivity
of the GO membranes cannot be explained solely by ionic-radius
based theories. They measured the electrical conductivities of
different permeate solutions and used this value to compare the
permeation rates of different salts. The potential applied to
measure the conductivities can affect ion permeation through
membranes.
[0008] Other publications (Y. Han, Z. Xu, C. Gao. Adv. Fund.
Mater. 23, 3693 (2013); M. Hu, B. Mi. Environ. Sci. Technol. 47,
3715 (2013); H. Huang et al. Chem. Comm. 49, 5963 (2013)) have
reported filtration properties of GO laminates and, although
results varied widely due to different fabrication and measurement
procedures, they reported appealing characteristics including
large water fluxes and notable rejection rates for certain salts.
Unfortunately, large organic molecules were also found to pass
through such GO filters. The latter observation is disappointing
and would considerably limit interest in GO laminates as molecular
sieves. In this respect, we note that the emphasis of these
studies was on high water rates that could be comparable to or
exceed the rates used for industrial desalination. Accordingly, a
high water pressure was applied and the GO membranes were
intentionally prepared as thin as possible, 10-50 nm thick. It may
be that such thin stacks contained holes and cracks (some may
appear after applying pressure), through which large organic
molecules could penetrate.
[0009] Recently, Joshi et al have described the use of graphene
oxide laminate membranes as size exclusion membranes (R. K. Joshi
et al., 2014, Science, 343, 752- 754). These membranes selectively
excluded solutes having a hydration radius greater than about 4.5
A. allowing solutes with a smaller radius to pass through.
Unfortunately many solutes which might be desirable to be able to
filter out, including for example NaCI, have hydration radii which
are below 4.5 A and thus cannot be excluded from passing through
the membrane. The GO laminate membranes described in Joshi et al
provide a water flux in the region of 2 L nr<2>h<"1>,
considerably lower than that typically obtained for commercial
filtration membranes.
BRIEF SUMMARY OF THE DISCLOSURE
[0010] In a first aspect of the invention is provided a method of
reducing the amount of one or more solutes in an aqueous mixture
to produce a liquid depleted in said solutes, the method
comprising:
a) contacting a first face of a graphene oxide laminate membrane
with the aqueous mixture comprising the one or more solutes;
b) recovering the liquid from or downstream from a second face of
the graphene oxide laminate membrane;
wherein the graphene oxide laminate membrane has a thickness
greater than about 100 nm and wherein the graphene oxide flakes of
which the membrane is comprised have an average oxygen:carbon
weight ratio in the range of from 0.2:1.0 to 0.5: 1.0 and wherein
the membrane comprises GO flakes and at least one cross-linking
agent. Thus, the membrane may comprise a cross-linking agent.
[0011] The cross-linked membranes used in the methods of the
invention exhibit considerably higher fluxes compared to GO
membranes which do not comprise cross- linking agents. Commercial
desalination membranes typically provide water fluxes ranges from
~ 1 L m-<2>h-<1>bar for seawater desalination to ~7 L
m-<2>h-<1>bar for high flux brackish water
desalination. GO laminate membranes which do not comprise a
cross-linking agent provide a water flux in the region of 2 L
nr<2>h<"1>with 25 bar pressure. The water flux of the
cross-linked GO laminate membranes used in the methods of the
invention are between 6 and 10 L nr<2>h<"1>with 25 bar
pressure, a significant improvement on the non-cross-linked
membranes. It would not be expected that reducing the size of the
pores in the hydrated membrane would lead to a higher flux.
Furthermore, the presence of a foreign material such as a
cross-linking agent in the GO membrane would be expected to impede
the passage of fluid through the membrane as it would be expected
to occupy some of the available voids of the material.
[0012] In a second aspect of the invention is provided a method of
reducing the amount of one or more solutes in an aqueous mixture
to produce a liquid depleted in said solutes, the method
comprising: a) contacting a first face of a graphene oxide
laminate membrane with the aqueous mixture comprising the one or
more solutes;
b) recovering the liquid from or downstream from a second face of
the graphene oxide laminate membrane;
wherein the graphene oxide laminate membrane comprises GO flakes
and graphene flakes. The membrane may also comprise at least one
cross-linking agent. The graphene flakes may be monolayer flakes
and/or few layer flakes.
[0013] It may be that the graphene oxide laminate membrane has a
thickness greater than about 100 nm and that the graphene oxide
flakes of which the membrane is comprised have an average
oxygen:carbon weight ratio in the range of from 0.2:1.0 to 0.5:
1.0.
[0014] The inventors have found that graphene/GO composite
membranes can be used as filtration membranes. Given that graphene
itself is impermeable it is perhaps surprising that such
composites can form an effective membrane.
[0015] The inventors have also found that by including
cross-linking agents or graphene in GO laminate membranes, the
expansion of the pores which usually occurs on hydration of GO
laminate membranes is reduced. This in turn can allow the membrane
to exclude smaller ions than would be excluded with GO laminate
membranes which do not comprise a cross-linking agent or graphene,
i.e. ions with hydration radii below 4.5 A. Additionally, or
alternatively, it can allow the membrane to be more effective at
excluding those smaller ions that can pass through. The extent to
which any given cross-linking agent constrains the membrane (i.e.
limits the expansion of the pores) on hydration of the membrane
varies depending on the identity of the cross-linking agent.
[0016] As the hydration radius at which an ion cannot pass through
the membrane is directly related to the d-spacing of the hydrated
laminate membrane, the size exclusion selectivity of these classes
of membranes can be tuned by selecting an appropriate cross-
linking agent and/or graphene. Thus, a membrane, and particularly
the cross-linking agent and/or graphene of which the membrane is
comprised, may be selected dependent on the size of the ions which
are being filtered.
[0017] The cross-linked membranes used in the methods of the
invention exhibit improved rejection of salts (e.g. NaCI) relative
to GO membranes which do not comprise a cross-linking agent.
[0018] Likewise, the graphene-GO (Gr-GO) composite membranes used
in the methods of the invention exhibit improved rejection of
salts (e.g. NaCI) relative to GO membranes which do not comprise
graphene. Gr-GO membranes do not exhibit a significant reduction
in water flux relative to GO membranes which do not comprise
graphene.
[0019] Indeed, for certain applications, the graphene GO composite
membranes are more effective at rejecting salts than cross-linked
GO membranes. Despite the fact that graphene is less effective
relative to many cross-linking agents at constraining the
expansion of membranes on hydration, the two dimensional structure
and more homogeneous distribution of graphene flakes through the
membrane than the cross-linking agents give rise to higher salt
rejection. It is believed that the areas of inhomogeneity in the
cross-linked GO membranes give rise to lower salt rejection than
expected based solely on the constraint the cross-linker applies
to the pores of the membrane.
[0020] It may be that the graphene flakes represent from 0.5 wt%
to 10 wt% of the flakes of which the graphene oxide laminate
membrane is comprised. It may be that the graphene flakes
represent from 1 wt% to 7.5 wt% of the flakes of which the
graphene oxide laminate membrane is comprised. It may be that the
graphene flakes represent from 2 wt% to 6 wt% of the flakes of
which the graphene oxide laminate membrane is comprised. The
inventors have found that the salt rejection properties of a GO
composite are improved by the inclusion of as little as about 1
wt% graphene. They have also found that when about 5wt% graphene
is included, the permeation rates of salts drop by around three
orders of magnitude.
[0021] Without wishing to be bound by theory, it is believed that
the inclusion of too much graphene in the GO laminate membranes
can make them too brittle for practical use and can also lead to a
loss of capillaries within the membranes meaning that water flux
can be lower for larger amounts of graphene.
[0022] It may be that greater than 50% by weight (e.g. greater
than 75% by weight, greater than 90% or greater than 98%) of the
graphene flakes have a diameter of less than 10 μηι. It may be
that greater than 50% by weight (e.g. greater than 75% by weight,
greater than 90% or greater than 98%) of the graphene flakes have
a diameter of greater than 50 nm. It may be that greater than 50%
by weight (e.g. greater than 75% by weight, greater than 90% or
greater than 98%) of the graphene flakes have a diameter of less
than 5 μηι. It may be that greater than 50% by weight (e.g.
greater than 75% by weight, greater than 90% or greater than 98%)
of the graphene flakes have a diameter of greater than 100 nm. It
may be that greater than 50% by weight (e.g. greater than 75% by
weight, greater than 90% or greater than 98%) of the graphene
flakes have a diameter of less than 1 μηι. It may be that greater
than 50% by weight (e.g. greater than 75% by weight, greater than
90% or greater than 98%) of the graphene flakes have a diameter of
less than 500 nm. [0023] It may be that greater than 50% by weight
(e.g. greater than 75% by weight, greater than 90% or greater than
98%) of the graphene has a thickness of from 1 to 10 atomic
layers. It may be that greater than 50% by weight (e.g. greater
than 75% by weight, greater than 90% or greater than 98%) of the
graphene has a thickness of from 1 to 5 molecular layers. Thus, it
may be that greater than 50% by weight (e.g. greater than 75% by
weight, greater than 90% or greater than 98%) of the graphene has
a thickness of from 1 to 3 molecular layers. It may be that
greater than 50% by weight (e.g. greater than 75% by weight,
greater than 90% or greater than 98%) of the graphene is single
layer graphene.
[0024] It may be that greater than 50% by weight (e.g. greater
than 75% by weight, greater than 90% or greater than 98%) of the
graphene oxide flakes have a diameter of less than 10 μηι. It may
be that greater than 50% by weight (e.g. greater than 75% by
weight, greater than 90% or greater than 98%) of the graphene
oxide flakes have a diameter of greater than 50 nm. It may be that
greater than 50% by weight (e.g. greater than 75% by weight,
greater than 90% or greater than 98%) of the graphene oxide flakes
have a diameter of less than 5 μηι. It may be that greater than
50% by weight (e.g. greater than 75% by weight, greater than 90%
or greater than 98%) of the graphene oxide flakes have a diameter
of greater than 100 nm. It may be that greater than 50% by weight
(e.g. greater than 75% by weight, greater than 90% or greater than
98%) of the graphene oxide flakes have a diameter of less than 2
μηι. It may be that greater than 50% by weight (e.g. greater than
75% by weight, greater than 90% or greater than 98%) of the
graphene oxide flakes have a diameter of less than 1 μηι. It may
be that greater than 50% by weight (e.g. greater than 75% by
weight, greater than 90% or greater than 98%) of the graphene
oxide flakes have a diameter of less than 500 nm. It may be that
greater than 50% by weight (e.g. greater than 75% by weight,
greater than 90% or greater than 98%) of the graphene oxide flakes
have a diameter of greater than 500 nm.
[0025] It may be that greater than 50% by weight (e.g. greater
than 75% by weight, greater than 90% or greater than 98%) of the
graphene oxide has a thickness of from 1 to 10 atomic layers. It
may be that greater than 50% by weight (e.g. greater than 75% by
weight, greater than 90% or greater than 98%) of the graphene
oxide has a thickness of from 1 to 5 molecular layers. Thus, it
may be that greater than 50% by weight (e.g. greater than 75% by
weight, greater than 90% or greater than 98%) of the graphene
oxide has a thickness of from 1 to 3 molecular layers. It may be
that greater than 50% by weight (e.g. greater than 75% by weight,
greater than 90% or greater than 98%) of the graphene oxide is
single layer graphene oxide. [0026] The solutes which are depleted
in the liquid have a hydration radius below a specific size
exclusion limit. It may be that the size exclusion limit is in the
range of from about 3.0 A to about 4.5 A. It may be that the size
exclusion limit is in the range of from about 3.25 A to about 4.25
A. It may be that the size exclusion limit is in the range of from
about 3.5 A to about 4.0 A.
[0027] The size exclusion limit depends in part on the average
spacing between the GO flakes, i.e. the height of the capillaries.
This average spacing can be measured indirectly, using x-ray
diffraction, as the d-spacing, which can be calculated from the
x-ray diffraction peaks using Bragg's law. The d-spacing of a
laminate membrane is effectively the sum of the thickness of the
GO flake and the distance between the GO flakes. The observed d-
spacing will be an average, the standard deviation of which will
depend on the width of the x-ray diffraction peaks. The width of
the x-ray diffraction peaks indicates how much variation there is
in the thickness of the GO flake and the distance between the GO
flakes. The x-ray diffraction peaks in cross-linked GO laminate
membranes tend to be broader than those in non-cross-linked
membranes, indicating that there is a greater variation in the
capillary size.
[0028] It may be that, when hydrated, the graphene oxide laminate
membrane has a d- spacing below 12 A. The d-spacing of the
hydrated graphene oxide laminate membrane may be below 11 A. The
d-spacing of the hydrated graphene oxide laminate membrane may be
below 10 A. The d-spacing of the hydrated graphene oxide laminate
membrane may be below 9 A. The d-spacing of the hydrated graphene
oxide laminate membrane may be below 8 A. The d-spacing of the
hydrated graphene oxide laminate membrane may be below 7 A.
[0029] The inventors have observed empirically a relationship
between the size exclusion limit and the d-spacing of the hydrated
membrane. The capillary size of the hydrated membrane is the
d-spacing minus the thickness of the GO flakes (typically between
3 and 3.5 A). The size exclusion limit is typically about half the
capillary size. Thus hydrated GO membranes with a d-spacing of
between 12 and 13 have a capillary size of between about 9 and 9.5
and a size exclusion cut off of about 4.5. Likewise, a hydrated
GO-polyAMPS cross-linked membrane has a d-spacing of about 9.1 A,
which would be expected to provide a capillary size of between
about 5.5 and 6 A and a size exclusion of about 3. It has been
observed that the GO-polyAMPS cross-linked membrane exhibits
excellent rejection of NaCI (the hydration radius of Na is 3.58
A).
[0030] In certain embodiments, the method is a process of
selectively reducing the amount of a first set of one or more
solutes in an aqueous mixture without significantly reducing the
amount of a second set of one or more solutes in the aqueous
mixture to produce a liquid depleted in said first set of solutes
but not depleted in said second set of solutes. In these
embodiments, the or each solute of the first set has a radius of
hydration greater than the size exclusion limit and the or each
solute of the second set has a radius of hydration less than the
size exclusion limit.
[0031] It may be that the method is continuous. Thus, steps a) and
b) may be carried out simultaneously or substantially
simultaneously. Steps a) and b) may also be carried out
iteratively in a continuous process to enhance enrichment or
iteratively in a batch process.
[0032] It may be that the aqueous mixture is permitted to pass
through the membrane by diffusion and / or it may be that a
pressure is applied. Preferably, pressure is applied.
[0033] Preferably, no electrical potential is applied across the
membrane. In principle, an electrical potential could be applied
to modify the transport of ions through the membrane.
[0034] The graphene oxide laminate membrane is optionally
supported on a porous material. This can provide structural
integrity. In other words, the graphene oxide flakes may
themselves form a layer e.g. a laminate which itself is associated
with a porous support such as a porous membrane to form a further
laminate structure. In this embodiment, the resulting structure is
a laminate of graphene flakes mounted on the porous support. In
one illustrative example, the graphene oxide laminate membrane may
be sandwiched between layers of a porous material. The use of a
porous support is particularly preferred where the graphene oxide
laminate membrane also comprises graphene. Such membranes can be
brittle.
[0035] It may be that the graphene oxide flakes of which the
laminate is comprised have an average oxygen:carbon weight ratio
in the range of from 0.2:1.0 to 0.5: 1.0, e.g. from 0.25: 1.0 to
0.45:1.0. Preferably, the flakes have an average oxygen:carbon
weight ratio in the range of from 0.3: 1.0 to 0.4: 1.0.
[0036] The GO flakes which form the membranes may have been
prepared by the oxidation of natural graphite.
[0037] The term "solute" applies to both ions and counter-ions,
and to uncharged molecular species present in the solution. Once
dissolved in aqueous media a salt forms a solute comprising
hydrated ions and counter-ions. The uncharged molecular species
can be referred to as "non-ionic species". Examples of non-ionic
species are small organic molecules such as aliphatic or aromatic
hydrocarbons (e.g. toluene, benzene, hexane, etc), alcohols (e.g.
methanol, ethanol, propanol, glycerol, etc), carbohydrates (e.g.
sugars such as sucrose), and amino acids and peptides. The
non-ionic species may or may not bind with water through hydrogen
bonds. As will be readily apparent to the person skilled in the
art, the term 'solute' does not encompass solid substances which
are not dissolved in the aqueous mixture. Particulate matter will
not pass through the membranes of the invention even if the
particulate is comprised of ions with small radii.
[0038] The term "hydration radius" refers to the effective radius
of the molecule when solvated in aqueous media.
[0039] The reduction of the amount one or more selected solutes in
the solution which is treated with the GO membrane of the present
invention may entail entire removal of the or each selected
solute. Alternatively, the reduction may not entail complete
removal of a particular solute but simply a lowering of its
concentration. The reduction may result in an altered ratio of the
concentration of one or more solutes relative to the concentration
of one or more other solutes. In cases in which salt is formed
from one ion having a hydration radius of larger than the size
exclusion limit and a counter-ion with a hydration radius below
the size exclusion limit, neither ion will pass through the
membrane of the invention because of the electrostatic attraction
between the ions. Thus, for example, if an NaCI solution were
passed through a membrane having a size exclusion limit of 3.5 A,
the amount of both the Na+ ions (hydration radius: 3.58 A) and the
CI- ions (hydration radius: 3.32 A) would be reduced, even though
the CI<">ions have a hydration radius below the size
exclusion limit.
[0040] The precise value of the size exclusion limit for any given
laminate membrane may vary depending on application. In the region
around the size exclusion limit, the degree of transmission
decreases by orders of magnitude and consequently the effective
value of the size exclusion limit depends on the amount of
transmission of solute that is acceptable for a particular
application.
[0041] The flakes of graphene oxide which are stacked to form the
laminate of the invention are usually monolayer graphene oxide.
However, it is possible to use flakes of graphene oxide containing
from 2 to 10 atomic layers of carbon in each flake. These
multilayer flakes are frequently referred to as "few-layer"
flakes. Thus the membrane may be made entirely from monolayer
graphene oxide flakes, from a mixture of monolayer and few-layer
flakes, or from entirely few-layer flakes. Ideally, the flakes are
entirely or predominantly, i.e. more than 75%w/w, monolayer
graphene oxide.
[0042] The graphene oxide laminates used in the methods of the
invention have the overall shape of a sheet-like material through
which solutes having a size below a certain size exclusion limit
may pass when the laminate is wet with an aqueous or aqueous-based
mixture optionally containing one or more additional solvents
(which may be miscible or immiscible with water). The solute may
only pass provided it is of sufficiently small size. Thus the
aqueous solution contacts one face or side of the membrane and
purified solution is recovered from the other face or side of the
membrane.
[0043] The method may involve a plurality of cross-linked graphene
oxide laminate membranes. These may be arranged in parallel (to
increase the flux capacity of the process/device) or in series
(where a reduction in the amount of one or more solute is achieved
by a single laminate membrane but that reduction is less than
desired).
[0044] The graphene oxide laminate membrane may have a thickness
greater than about 100 nm, e.g. greater than about 500 nm, e.g. a
thickness between about 500 nm and about 100 μηι. The graphene
oxide laminate membrane may have a thickness up to about 50 μηι.
The graphene oxide laminate membrane may have a thickness greater
than about 1 μηι, e.g. a thickness between 1 μηι and 15 μηι. Thus,
the graphene oxide laminate membrane may have a thickness of about
5 μηι.
[0045] A cross linking agent is a substance which bonds with GO
flakes in the laminate. The cross linking agent may form hydrogen
bonds with GO flakes or it may form covalent bonds with GO flakes.
Examples (which are included in some embodiments of the invention
but which may be specifically excluded from other embodiments of
the invention) include diamines (e.g. ethyl diamine, propyl
diamine, phenylene diamine), polyallylamines and imidazole.
Without wishing to be bound by theory, it is believed that these
are examples of crosslinking agents which form hydrogen bonds with
GO flakes. Other examples include borate ions and polyetherimides
formed from capping the GO with polydopamine. Examples of
appropriate cross linking systems can be found in Tian et al,
(Adv. Mater. 2013, 25, 2980-2983), An et al (Adv. Mater. 2011 ,
23, 3842-3846), Hung et al (Cross-linking with Diamine monomers to
Prepare Composite Graphene Oxide- Framework Membranes with Varying
d-Spacing; Chemistry of Materials, 2014) and Park et al(Graphene
Oxide Sheets Chemically Cross-Linked by polyallylamine; J. Phys.
Chem. C; 2009).
[0046] The crosslinking agent may be a polymer. The polymer may be
interspersed throughout the membrane. It may occupy the spaces
between graphene oxide flakes, thus providing interlayer
crosslinking. Examples (which are included in some embodiments of
the invention but which may be specifically excluded from other
embodiments of the invention) include PVA (see for example Li et
al Adv. Mater. 2012, 24, 3426-3431), poly(4- styrenesulfonate),
Nafion, carboxymethyl cellulose, Chitosan, polyvinyl pyrrolidone,
polyaniline etc. A preferred polymer is
poly(2-acrylamido-2-methyl-1-propanesulfonic acid. It may be that
the polymer is water soluble. Alternatively, it may be that the
polymer is not water soluble. [0047] The cross-linking agent may
be a charged polymer, e.g. one which comprises sulfonic acids or
other ionisable functional groups. Exemplary charged polymers
include poly(4-styrenesulfonate), Nafion and
poly(2-acrylamido-2-methyl-1-propanesulfonic acid.
[0048] The cross-linking agent (e.g. polymer or charged polymer)
may be present in an amount from about 0.1 to about 50 wt%, e.g.
from about 5 to about 45 wt%. Thus, the GO laminate may comprise
from about 2 to about 25 wt% cross-linking agent (e.g. polymer or
charged polymer), the GO laminate may comprise up to about 20 wt%
cross-linking agent (e.g. polymer or charged polymer).
[0049] The graphene flakes may be monolayer graphene flakes. They
may be few-layer (i.e. 2-10 atomic layers, e.g. 3-7 atomic layers)
graphene flakes. The graphene may be a reduced graphene oxide or
partially oxidized graphene. Preferably, however, it is pristine
graphene. The graphene may be pristine graphene with small holes
in it. The defects in reduced graphene oxide or partially oxidized
graphene or holes in pristine graphene can lead to higher fluxes.
[0050] The GO laminates may comprise other inorganic materials,
e.g. other two dimensional materials, such as hBN, mica. The
presence of mica, for example can slightly improve the mechanical
properties of the GO laminate.
[0051] It may be that, if present, the porous support is an
inorganic material. Thus, the porous support (e.g. membrane) may
comprise a ceramic. Preferably, the support is alumina, zeolite,
or silica. In one embodiment, the support is alumina. Zeolite A
can also be used. Ceramic membranes have also been produced in
which the active layer is amorphous titania or silica produced by
a sol-gel process.
[0052] It may be that, if present, the porous support is a
polymeric material. Thus, the porous support may thus be a porous
polymer support, e.g. a flexible porous polymer support.
Preferably it is PES, PTFE, PVDF or Cyclopore™ polycarbonate. In
an embodiment, the porous support (e.g. membrane) may comprise a
polymer. In an embodiment, the polymer may comprise a synthetic
polymer. These can be used in the invention. Alternatively, the
polymer may comprise a natural polymer or modified natural
polymer. Thus, the polymer may comprise a polymer based on
cellulose. The polymer support may be derived from a charged
polymer such as one which contains sulfonic acids or other
ionisable functional groups.
[0053] It may be that, if present, the porous support (e.g.
membrane) may comprise a carbon monolith.
[0054] In an embodiment, the porous support layer has a thickness
of no more than a few tens of μηι, and ideally is less than about
100 μηι. Preferably, it has a thickness of 50 μηι or less, more
preferably of 10 μηι or less, and yet more preferably is less 5
μηι. In some cases it may be less than about 1 μηι thick though
preferably it is more than about 1 μηι.
[0055] Preferably, the thickness of the entire membrane (i.e. the
graphene oxide laminate and the support, if present) is from about
1 μηι to about 200 μηι, e.g. from about 5 μηι to about 50.
[0056] The porous support should be porous enough not to interfere
with water transport but have small enough pores that graphene
oxide platelets cannot enter the pores. Thus, the porous support
must be water permeable. In an embodiment, the pore size must be
less than 1 μηι. In an embodiment, the support has a uniform
pore-structure. Examples of porous membranes with a uniform pore
structure are electrochemically manufactured alumina membranes
(e.g. those with the trade names: Anopore™, Anodisc™).
[0057] The one or more solutes can be ions and/or they could be
neutral organic species, e.g. sugars, hydrocarbons etc. Where the
solutes are ions they may be cations and/or they may be anions.
[0058] In certain preferred embodiments, the solutes are
Na<+>ions and/or CI<">ions. Thus the method may be a
method of desalination (i.e. a method of reducing the amount of
NaCI in an aqueous mixture).
[0059] In a third aspect of the invention is provided a method of
reducing the amount of one or more predetermined solutes having a
hydration radius in the range of from about 3.5 A to about 4.5 A
in an aqueous mixture to produce a liquid depleted in the
predetermined solutes, the method comprising;
a) determining the identity of one or more solutes in the aqueous
mixture which are to be selected for exclusion by the membrane;
b) correlating the required d-spacing in the graphene oxide
membrane with the hydration radius of the or each predetermined
solute;
c) forming a graphene oxide laminate membrane comprising GO flakes
and also comprising monolayer or few layer graphene flakes and/or
at least one cross linking agent and having a reduced d-spacing
relative to a membrane which does not comprise the cross-linking
agent;
d) contacting a first face of a graphene oxide laminate membrane
with the aqueous mixture comprising one or more solutes; and
e) recovering the liquid from or downstream from a second face of
the membrane. [0060] It may be that steps d) and e) are performed
continuously. Thus, steps d) and e) may be carried out
simultaneously or substantially simultaneously.
[0061] In a fourth aspect of the invention is provided a method of
tuning the d-spacing of a cross-linked graphene oxide laminate
size exclusion filtration membrane, the method comprising:
a) selecting at least one cross-linking agent and/or monolayer or
few layer graphene flakes which provides a membrane having a
desired capillary size when the membrane is hydrated; and
b) forming a graphene oxide laminate membrane comprising GO flakes
and also comprising monolayer or few layer graphene flakes and/or
the at least one cross linking agent.
[0062] In a fifth aspect of the invention is provided a method of
limiting the d-spacing of a hydrated graphene oxide laminate size
exclusion filtration membrane to below 12 A, the method
comprising:
forming a graphene oxide laminate membrane comprising GO flakes
and also comprising monolayer or few layer graphene flakes and/or
at least one cross linking agent.
[0063] The cross-linking agent is solubilised by reference to
cross-linking agents that have been determined experimentally to
provide the required d-spacing or less.
[0064] In a sixth aspect of the invention is provided the use of
monolayer or few layer graphene flakes and/or at least one cross
linking agent to limit the d-spacing of a hydrated graphene oxide
laminate size exclusion filtration membrane to below 12 A.
[0065] Suitable cross-linking agents and the means for determining
them are described herein.
[0066] In a seventh aspect of the invention is provided a graphene
oxide laminate membrane comprising GO flakes and a charged polymer
(e.g. poly(2-acrylamido-2-methyl- 1-propanesulfonic acid) as a
cross-linking agent. The charged polymer may be one which
comprises sulfonic acids or other ionisable functional groups.
Exemplary charged polymers include poly(4-styrenesulfonate),
Nafion and poly(2-acrylamido-2-methyl-1- propanesulfonic acid.
[0067] In an eighth aspect of the invention is provided a graphene
oxide laminate membrane comprising GO flakes and at least one
cross linking agent and having, when hydrated, a reduced pore size
relative to a hydrated graphene oxide membrane which does not
comprise the cross-linking agent, and wherein the pore size in the
hydrated membrane is operative to substantially exclude at least
the passage of solutes having a hydration radius in the range of
from about 3.5A to about 4.5A when present in an aqueous mixture.
[0068] In a ninth aspect of the invention is provided a graphene
oxide laminate membrane comprising GO flakes and monolayer or few
layer graphene flakes.
[0069] In a tenth aspect of the invention is provided a method of
producing a graphene oxide laminate membrane comprising GO flakes
and graphene flakes, the method comprising:
a) providing a suspension of graphite flakes and graphite oxide
flakes in an aqueous medium;
b) subjecting the graphite flakes and graphite oxide flakes in the
aqueous medium to energy to obtain an aqueous suspension
comprising graphene flakes and graphene oxide flakes;
c) optionally removing any graphite, graphite oxide or undesired
few-layered graphene/graphene oxide flakes from the suspension;
and
d) filtering the suspension through a porous material to provide a
graphene oxide laminate membrane comprising GO flakes and graphene
flakes, the laminate membrane being supported on the porous
material.
[0070] The energy applied in step (b) may be sonic energy. The
sonic energy may be ultrasonic energy. It may be delivered in
using a bath sonicator or a tip sonicator.
Alternatively the energy may be a mechanical energy, e.g. shear
force energy or grinding. The particles may be subjected to energy
(e.g. sonic energy) for a length of time from 15 min to 1 week,
depending on the properties and proportions (flake diameter and
thickness) desired. The particles may be subjected to energy (e.g.
sonic energy) for a length of time from 1 to 4 days.
[0071] Where the desired laminate membrane also comprises
cross-linkng agents, these will be present in the aqueous medium
prior to filtration. They may be present in the suspension of
graphite and graphite oxide or they may be added after step b) or,
if present, step c).
[0072] The term 'aqueous medium' can be understood to mean a
liquid which contains water, e.g. which contains greater than 20%
by volume water. The aqueous medium may contain more than 50% by
volume water, e.g. more than 75% by volume water or more than 95%
by volume water. The aqueous medium may also comprise solutes or
suspended particles and other solvents (which may or may not be
miscible with water). The aqueous medium may comprise additives
which may be ionic, organic or amphiphillic. Examples of such
additives include surfactants, viscosity modifiers, pH modifiers,
iconicity modifiers, and dispersants. It may be however that the
aqueous medium consists essentially of water, graphite and
graphite oxide and optionally one or more cross-linking agents
[0073] The step of reducing the amount of multilayered particles
in the suspension may comprise using a centrifuge.
[0074] Graphene oxide is able to stabilise graphene flakes in an
aqueous medium, similarly to the action of various surfactants.
Thus, once the graphite oxide has been exfoliated, the thus formed
graphene oxide flakes encourage the exfoliation of the graphite
into graphene flakes and/or stabilise the graphene flakes ocne
they have been exfoliated. Smaller GO flakes are more effective
dispersants than larger GO flakes (e.g. less than 1 μηι or less
than 500 nm).
[0075] Typically, the exfoliation of graphite oxide is more
efficient than the exfoliation of graphite. Thus, the starting
suspension might contain more graphite than graphite oxide.
Indeed, the ratio of graphite to graphite oxide may be larger than
that desired in the product membrane. For example, the inventors
have found that a weight ratio of 9:1 graphite: graphite oxide
mixture gives rise to a membrane which is 5.5 wt% graphene.
[0076] In an eleventh aspect of the invention is provided a
filtration device comprising a membrane of the seventh, eighth or
ninth aspects of the invention. The filtration device may be a
filter assembly or it may be a removable and replaceable filter
for use in a filter assembly.
[0077] In certain embodiments, it may be that the cross-linking
agent is not selected from: diamine; pollyallylamine; imidazole;
borate ions; polyetherimides formed from capping GO with
polypodamine; PVA; poly(4-styrenesulfonate); Nafion, caboxymethyl
cellulose; chitosan; polyvinyl pyrrolidone; and polyaniline. This
applies in particular to the third to eighth aspects of the
invention.
[0078] In any of the third to tenth aspects of the invention, it
may be that the graphene oxide laminate membrane has a thickness
greater than about 100 nm. Likewise, it may be that the graphene
oxide flakes of which the laminate is comprised have an average
oxygen:carbon weight ratio in the range of from 0.2: 1.0 to 0.5:
1.0.
[0079] Where not mutually exclusive, any of the embodiments
described above in relation to the first and/or second aspects of
the invention apply equally to one or more of the second to
eleventh aspects of the invention.
BRIEF DESCRIPTION OF THE DRAWINGS
[0080] Embodiments of the invention are further described
hereinafter with reference to the accompanying drawings, in
which:
Figure 1 shows the x-ray diffraction peaks for selected
cross-linked and non-cross-linked laminate membranes before
hydration and the corresponding observed d-spacings.
Figure 2 shows the d-spacing of selected cross-linked and
non-cross-linked laminate membranes both before (Bf Hyd) and
after (Af Hyd) hydration.
Figure 3 shows the water flux of selected cross-linked and
non-cross-linked laminate membranes (with an applied pressure of
25 bar).
Figure 4 shows the NaCI rejection of selected cross-linked
and non-cross-linked laminate membranes as an average across all
measurements.
Figure 5 shows the NaCI rejection of selected cross-linked
and non-cross-linked laminate membranes in terms of the value
obtained for each measurement.
Figure 6 shows the MgC rejection of selected cross-linked
and non-cross-linked laminate membranes.
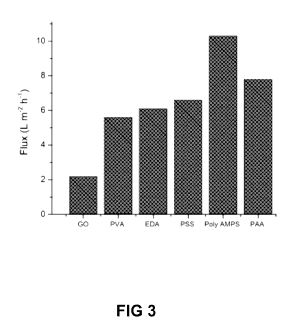
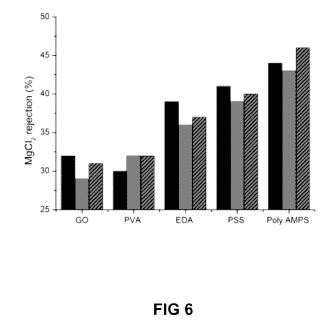
DETAILED DESCRIPTION
[0081] The present invention involves the use of graphene oxide
laminate membranes. The graphene oxide laminates and laminate
membranes of the invention comprise stacks of individual graphene
oxide flakes, in which the flakes are predominantly monolayer
graphene oxide. Although the flakes are predominantly monolayer
graphene oxide, it is within the scope of this invention that some
of the graphene oxide is present as two- or few-layer graphene
oxide. Thus, it may be that at least 75% by weight of the graphene
oxide is in the form of monolayer graphene oxide flakes, or it may
be that at least 85% by weight of the graphene oxide is in the
form of monolayer graphene oxide flakes (e.g. at least 95 %, for
example at least 99% by weight of the graphene oxide is in the
form of monolayer graphene oxide flakes) with the remainder made
up of two- or few- layer graphene oxide. Without wishing to be
bound by theory, it is believed that water and solutes pass
through capillary-like pathways formed between the graphene oxide
flakes by diffusion and that the specific structure of the
graphene oxide laminate membranes leads to the remarkable
selectivity observed as well as the remarkable speed at which the
ions permeate through the laminate structure.
[0082] Graphene oxide flakes are two dimensional heterogeneous
macromolecules containing both hydrophobic 'graphene' regions and
hydrophilic regions with large amounts of oxygen functionality
(e.g. epoxide, carboxylate groups, carbonyl groups, hydroxyl
groups)
[0083] In one illustrative example, the graphene oxide laminate
membranes are made of impermeable functionalized graphene sheets
that have a typical size L «1 μηι and the interlayer separation,
d, sufficient to accommodate a mobile layer of water.
[0084] The solutes to be removed from aqueous mixtures in the
methods of the present invention may be defined in terms of their
hydrated radius. Below are the hydrated radii of some exemplary
ions and molecules.
Table 1
[0085] The hydrated radii of many species are available in the
literature. However, for some species the hydrated radii may not
be available. The radii of many species are described in terms of
their Stokes radius and typically this information will be
available where the hydrated radius is not. For example, of the
above species, there exist no literature values for the hydrated
radius of propanol, sucrose, glycerol and PTS<4">. The
hydrated radii of these species which are provided in the table
above have been estimated using their Stokes/crystal radii. To
this end, the hydrated radii for a selection of species in which
this value was known can be plotted as a function of the Stokes
radii for those species and this yields a simple linear
dependence. Hydrated radii for propanol, sucrose, glycerol and
PTS<4">were then estimated using the linear dependence and
the known Stokes radii of those species.
[0086] There are a number of methods described in the literature
for the calculation of hydration radii. Examples are provided in
'Determination of the effective hydrodynamic radii of small
molecules by viscometry'; Schultz and Soloman; The Journal of
General
Physiology; 44; 1 189-1 199 (1963); and 'Phenomenological Theory
of Ion Solvation'; E. R. Nightingale. J. Phys. Chem. 63, 1381
(1959).
[0087] The term 'aqueous mixture' refers to any mixture of
substances which comprises at least 10% water by weight. It may
comprise at least 50% water by weight and preferably comprises at
least 80% water by weight, e.g. at least 90% water by weight. The
mixture may be a solution, a suspension, an emulsion or a mixture
thereof. Typically the aqueous mixture will be an aqueous solution
in which one or more solutes are dissolved in water. This does not
exclude the possibility that there might be particulate matter,
droplets or micelles suspended in the solution. Of course, it is
expected that the particulate matter will not pass through the
membranes of the invention even if it is comprised of ions with
small radii.
[0088] Particularly preferred solutes for removing from water
include hydrocarbons and oils, biological material, dyes, organic
compounds (including halogenated organic compounds), complex ions,
NaCI, heavy metals, ethanol, chlorates and perchlorates and
radioactive elements.
[0089] The graphene oxide or graphite oxide for use in this
application can be made by any means known in the art. In a
preferred method, graphite oxide can be prepared from graphite
flakes (e.g. natural graphite flakes) by treating them with
potassium
permanganate and sodium nitrate in concentrated sulphuric acid.
This method is called Hummers method. Another method is the Brodie
method, which involves adding potassium chlorate (KCIO3) to a
slurry of graphite in fuming nitric acid. For a review see, Dreyer
et al. The chemistry of graphene oxide, Chem. Soc. Rev., 2010, 39,
228-240.
[0090] Individual graphene oxide (GO) sheets can then be
exfoliated by dissolving graphite oxide in water or other polar
solvents with the help of ultrasound, and bulk residues can then
be removed by centrifugation and optionally a dialysis step to
remove additional salts.
[0091] In a specific embodiment, the graphene oxide of which the
graphene oxide laminate membranes of the invention are comprised
is not formed from wormlike graphite. Worm-like graphite is
graphite that has been treated with concentrated sulphuric acid
and hydrogen peroxide at 1000 °C to convert graphite into an
expanded "worm-like" graphite. When this worm-like graphite
undergoes an oxidation reaction it exhibits a higher increase the
oxidation rate and efficiency (due to a higher surface area
available in expanded graphite as compared to pristine graphite)
and the resultant graphene oxide contains more oxygen functional
groups than graphene oxide prepared from natural graphite.
Laminate membranes formed from such highly functionalized graphene
oxide can be shown to have a wrinkled surface topography and
lamellar structure (Sun et al,; Selective Ion Penetration of
Graphene Oxide Membranes; ACS Nano 7, 428 (2013) which differs
from the layered structure observed in laminate membranes formed
from graphene oxide prepared from natural graphite. Such membranes
do not show fast ion permeation of small ions and a selectivity
which is substantially unrelated to size (being due rather to
interactions between solutes and the graphene oxide functional
groups) compared to laminate membranes formed from graphene oxide
prepared from natural graphite.
[0092] The preparation of graphene oxide laminate supported on a
porous membrane can be achieved using filtration, spray coating,
casting, dip coating techniques, road coating, inject printing, or
any other thin film coating techniques
[0093] For large scale production of supported graphene based
membranes or sheets it is preferred to use spray coating, road
coating or inject printing techniques. One benefit of spray
coating is that spraying GO solution in water on to the porous
support material at an elevated temperature produces a large
uniform GO film.
[0094] Graphite oxide consists of micrometer thick stacked
graphite oxide flakes (defined by the starting graphite flakes
used for oxidation, after oxidation it gets expanded due to the
attached functional groups) and can be considered as a
polycrystalline material.
Exfoliation of graphite oxide in water into individual graphene
oxide flakes was achieved by the sonication technique followed by
centrifugation at 10000 rpm to remove few layers and thick flakes.
Graphene oxide laminates were formed by restacking of these single
or few layer graphene oxides by a number of different techniques
such as spin coating, spray coating, road coating and vacuum
filtration.
[0095] Graphene oxide membranes according to the invention consist
of overlapped layers of randomly oriented single layer graphene
oxide sheets with smaller dimensions (due to sonication). These
membranes can be considered as centimetre size single crystals
(grains) formed by parallel graphene oxide sheets. Due to this
difference in layered structure, the atomic structure of the
capillary structure of graphene oxide membranes and graphite oxide
are different. For graphene oxide membranes the edge functional
groups are located over the non-functionalised regions of another
graphene oxide sheet while in graphite oxide mostly edges are
aligned over another graphite oxide edge. These differences
unexpectedly may influence the permeability properties of graphene
oxide membranes as compared to those of graphite oxide.
[0096] A layer of graphene consists of a sheet of
sp<2>-hybridized carbon atoms. Each carbon atom is
covalently bonded to three neighboring carbon atoms to form a
'honeycomb' network of tessellated hexagons. Carbon nanostructures
which have more than 10 graphene layers (i.e. 10 atomic layers;
3.5 nm interlayer distance) generally exhibit properties more
similar to graphite than to mono-layer graphene. Thus, throughout
this specification, the term graphene is intended to mean a carbon
nanostructure with up to 10 graphene layers. A graphene layer can
be considered to be a single sheet of graphite.
[0097] In the context of this disclosure the term graphene is
intended to encompass both pristine graphene (i.e.
un-functionalised or substantially un-functionalised graphene) and
reduced graphene oxide. When graphene oxide is reduced a graphene
like substance is obtained which retains some of the oxygen
functionality of the graphene oxide. It may be however that the
term 'graphene' is excludes both graphene oxide and reduced
graphene oxide and thus is limited to pristine graphene. All
graphene contains some oxygen, dependent on the oxygen content of
the graphite from which is it derived. It may be that the term
'graphene' encompasses graphene that comprises up to 10% oxygen by
weight, e.g. less than 8% oxygen by weight or less than 5% oxygen
by weight. .
[0098] Throughout the description and claims of this
specification, the words "comprise" and "contain" and variations
of them mean "including but not limited to", and they are not
intended to (and do not) exclude other moieties, additives,
components, integers or steps. Throughout the description and
claims of this specification, the singular encompasses the plural
unless the context otherwise requires. In particular, where the
indefinite article is used, the specification is to be understood
as contemplating plurality as well as singularity, unless the
context requires otherwise.
[0099] Features, integers, characteristics, compounds, chemical
moieties or groups described in conjunction with a particular
aspect, embodiment or example of the invention are to be
understood to be applicable to any other aspect, embodiment or
example described herein unless incompatible therewith. All of the
features disclosed in this specification (including any
accompanying claims, abstract and drawings), and/or all of the
steps of any method or process so disclosed, may be combined in
any combination, except combinations where at least some of such
features and/or steps are mutually exclusive. The invention is not
restricted to the details of any foregoing embodiments. The
invention extends to any novel one, or any novel combination, of
the features disclosed in this specification (including any
accompanying claims, abstract and drawings), or to any novel one,
or any novel combination, of the steps of any method or process so
disclosed.
[00100] The reader's attention is directed to all papers and
documents which are filed concurrently with or previous to this
specification in connection with this application and which are
open to public inspection with this specification, and the
contents of all such papers and documents are incorporated herein
by reference.
EXAMPLE 1 - Cross-linked GO laminate membranes
[00101] Graphite oxide was prepared from natural graphite through
modified Hummer's method using sulphuric acid and potassium
permanganate. The graphite oxide was then dispersed in water by
ultrasonication to obtain the stable aqueous graphene oxide (GO)
dispersion. The unexfoliated graphite oxide and few layer graphene
oxide flakes were removed by centrifugation and the supernatant
containing the single layer GO sheets was used for the membrane
preparation. Then, a cross linker selected from poly vinyl alcohol
(PVA), ethylenediamine (EDA), poly (styrene-4-sulfonate) (PSS),
poly Allylamine (PAA) and
poly(2-acrylamido-2-methyl-1-propanesulfonic acid) (poly AMPS)
(20% with respect to the weight of the GO present in solution) was
dissolved in GO suspension and left for overnight stirring at room
temperature. By adjusting the volume of each solution, GO-PVA,
GO-EDA, GO-PSS, GO-PAA and GO-polyAMPS membranes of thicknesses ~
500 nm, were prepared on the polyethersulfone (PES) membrane
(diameter of 47 mm with pore size ~ 0.2 μηι) using vacuum
filtration. The membranes were dried in a vacuum desiccator in
prior to use for the pressure filtration experiments.
[00102] X-ray diffraction (XRD) was used to measure the
inter-layer d spacing (capillary width) of the GO membranes. The
d-spacing values are calculated from the peak position in XRD
pattern using Bragg's law ηλ = 2d sinO. For XRD experiments,
GO-PVA, GO-EDA, GO-PSS, GO-PAA and GO-polyAMPS membranes (of
thickness ~ 5 μηι) are prepared by vacuum filtration of each
solution through Anodisc alumina membranes with a pore size of
0.02 μηι. These membranes were dried under vacuum to peel a free
standing GO membrane with different linker molecules for the XRD
measurements. Bruker D8-Discover X-ray diffractometer was used to
estimate the d-spacing of the fabricated free standing membranes
in both dry and wet states. XRD pattern (5 < 2Θ < 25) of the
each free standing membrane was obtained at room temperature and
room humidity and left these membranes in water for 24 hrs.
Further, the XRD measurements were conducted on soaked membranes
in the same 2Θ range to estimate the swelling effect. From the XRD
measurements of all the GO membranes with different linker
molecules, GO-polyAMPS membrane has shown very small increase in
the d-spacing from 8.6 A (in dry state) to 9.1 A (in wet state).
[00103] In the present study, we have used Sterlitech HP4750
stirred cell for pressure filtration experiments. Various GO
membranes with different linkers prepared on the PES were placed
in the pressure filtration cell with a porous metal support and
performed the pressure filtration experiments for 2 mg/ml MgC and
NaCI solutions by applying 26 bar pressure using a compressed gas
cylinder. The solution permeated through the membranes was
collected from the permeate tube fixed to the pressure filtration
cell. Among all the GO membranes with different linkers,
GO-polyAMPS membrane has shown flux rate of 10 L
nr<2>h<"1>with a salt rejection ~ 50%. The data
obtained is shown in figures 1 to 6.
EXAMPLE 2 - Graphene oxide/graphene composite laminate
membranes
[00104] 250 mg of graphite oxide (prepared as in Example 1) and
125 mg of pristine graphite powder were sonicated in 250 ml of Dl
water for 24 hrs to prepare a Gr-GO dispersion. The Gr-GO
suspension was then centrifuged at 2500 rpm to remove the
unexfoliated graphite oxide and graphite particles with the
supernatant containing the mono and few layers of GO and graphene
flakes (this sample was denoted as denoted as Gr-GO-2500). In this
method, graphene oxide helps exfoliated graphene to disperse in
water to form a stable aqueous suspension.
[00105] Gr-GO membranes were prepared by vacuum filtration of
Gr-GO dispersion through an Anodisc membrane filter (47 mm in
diameter, 0.2 mm pore size) similarly to the method described in
Example 1. Gr-GO membranes with Anodisc support were glued onto
copper plates which exposes an effective area of ~ 1 cm<2>of
the membrane. The copper plate was then placed in a permeation
setup containing the feed and permeates compartments. In a typical
experiment, feed compartment filled with 1 M aqueous solution of
various salts and the permeate compartment was filled with Dl
water and kept undisturbed for 24 hrs. Inductively coupled plasma
optical emission spectroscopy (ICP- OES) was used to find the ion
species concentration in the permeate cell. Also these results
were cross checked by carefully weighing the left over material
after the evaporation of water in permeate compartment. It is
found that the permeation rate for Mg<+2>and Na<+>ions
for the Gr-GO membrane is ~ 2 x 10<"3>and 3 x
10<"3>mol/h/m<2>which is 1000 times smaller when
compared to that of the GO laminate membrane which does not
comprise graphene or a cross-linking agent. In another permeation
experiment with GO- polyAMPS, the permeation rate of
Mg<+2>ions found to be ~ 1 x 10<"2>mol/h/m<2>.
[00106] The amount of graphene present in the Gr-GO suspension can
be controlled by centrifuging the dispersion obtained from
sonication of the graphite and graphite oxide mixture at differing
speeds. Thus, samples obtained from sonication of the graphite and
graphite oxide mixture as described above were centrifuged at
5000, 7500 and 10000 rpm and the resultant suspension was formed
into a laminate membrane as described above. From the permeation
experiments with Gr-GO membranes made of the Gr-GO dispersion
centrifuged at 5000, 7500 and 10000 rpm (denoted as Gr-GO-5000,
Gr-GO-7500 and Gr- GO-10000), it was found that permeation rate of
the Mg<+2>ions (given in the table below) increased for
Gr-GO membranes prepared with the Gr-GO dispersion centrifuged at
higher speeds. Permeation rate of Mg<+2>ions in the
GO/graphene-10000 is 10 times more than that of in the
GO/graphene-2500. It is expected that lower centrifugation rates
result in a higher proportion of the membrane containing few layer
graphene too.
EXAMPLE 3 - Graphene oxide/graphene composite laminate
membranes
[00107] Further, four different concentrations of Gr-GO aqueous
dispersions were prepared by exfoliating the graphite flakes and
graphite oxide in the weight ratio (graphite oxide/graphite) of 1
: 1 , 1 :2, 1 :5 and 1 :9. 0.175 g of graphite oxide was sonicated
in 120 ml deionised water along with different weights of graphite
flakes varying as 0.175 g, 0.35 g, 0.875 g and 1.575 g for 50 hrs.
Supernatant of the resulting dispersion was collected after few
hours to avoid the unexfoliated graphite and unstable aggregates
which settles down gradually. Subsequently, the supernatant was
centrifuged twice for 25 mins at 2500 g to obtain the homogenous
Gr-GO aqueous dispersion containing mono and few layers GO and
graphene flakes. The Gr-GO membranes were prepared by vacuum
filtration of Gr- GO dispersion through an Anodisc membrane filter
(47 mm diameter, 0.02 μηι pore size) and dried in a vacuum
desiccator.
[00108] For the permeation experiments, Gr-GO membranes with
Anodisc support were glued onto copper plates in such a way that
an effective area of ~ 1 cm<2>of the membrane is exposed
[15]. A typical permeation experiment was carried out for 24 hrs
by fixing the membrane attached copper plate in a permeation setup
where feed compartment filled with 1 M aqueous solution of various
salts (KCI, NaCI, LiCI and MgC ) and the permeate compartment was
filled with deionised water. Inductively coupled plasma optical
emission spectroscopy (ICP-OES) was used to find the ion species
concentration in the permeate cell and these results were cross
checked by carefully weighing the left over material after the
evaporation of water in permeate compartment.
[00109] Fig. 8a shows the optical photograph of 100 μg/ml
concentrated GO and Gr-GO aqueous colloidal suspensions with
increasing wt% of graphene (from left to right). The pale brown
coloured GO suspension gradually turns into dark with increasing
initial amount of graphite starting material which suggests the
increased amount of exfoliated graphene in Gr-GO dispersions in
the case of higher initial graphite content. Weight of graphite
oxide is kept constant and varied the initial weight of graphite
flakes for preparation of each solution in order to estimate the
actual wt% of graphene exfoliated into GO suspension. Fig. 8b
shows the concentration and actual wt% of exfoliated graphene in
GO and Gr-GO dispersions as a function of initial graphite
oxide/graphite weight ratio. Three membranes (GO and Gr-GO)
prepared from the known amount of volume of each dispersion were
carefully weighed using '^g" precision microbalance to determine
the concentration of GO and Gr-GO dispersions. Subsequently,
actual wt% of exfoliated graphite in the different Gr- GO
dispersion is estimated from the concentration values and found
that ~ 5.5 wt%, 4.2 wt%, 2.2 wt% and 1.3 wt% of exfoliated
graphene (with respect to the weight of GO) is present in the
membranes made from the Gr-GO dispersions of 1 :9, 1 :5, 1 :2 and
1 : 1 initial graphite oxide/graphite ratio, respectively.
[001 10] X-ray diffraction technique has been used to analyse the
changes in the interlayer spacing of GO and Gr-GO membranes in
both dry and wet states. In the dry state, both pristine GO and
Gr-GO membranes show similar (001) diffraction peak at ~ 10.5±0.5°
indicating similar laminar structures for both the membranes. To
determine the swelling behaviour, GO and Gr-GO membranes were
soaked for a day in deionised water. As expected, the interlayer
spacing (-8.4 A in dry state) of GO membrane increased to 14 A
after soaking. In contrast to the GO membranes, Gr-GO membranes
having a higher wt% of exfoliated graphene flakes have shown less
swelling. For example, the interlayer spacing of Gr-GO membranes
with 5.5 wt% and 2.2 wt% of exfoliated graphene is respectively ~
10.3 A and 11.4 A in the wet state. This indicates that
incorporation of exfoliated graphene in the GO membrane controls
the swelling of GO membrane by controlling the amount of water in
the interlayer space. Without wishing to be bound by theory, this
could be due to the more hydrophobic nature of exfoliated graphene
which lowers the amount of water in the interlayer spaces of the
membrane.
[001 11] Homogeneity of the Gr-GO membranes was further confirmed
by the SEM investigations. Fig. 7a and 7b show the cross-sectional
and in-plain SEM image of Gr-GO membrane respectively.
Distribution of exfoliated graphite (flaky features in Fig.7b) in
the membrane is found to be very uniform and they are shown to be
assembled in a layered structure. Fig. 7c shows the schematic
structure of layered structure of Gr-GO membrane.
[001 12] Fig. 8d summarizes the permeation rate for different ions
(K<+>, Na<+>, Li<+>and Mg<+2>) through the
GO and Gr-GO membranes made from 1 :2 and 1 :9 dispersions. From
Fig. 8d, it is apparent that the value of permeation rate observed
for Mg<+2>ions through Gr-GO membrane made of 1 :9
dispersion is ~ 1000 times smaller than the permeation rate
through pristine GO membrane. This can be explained by the lesser
swelling effect in 1 :9 Gr-GO membrane with respect to the
pristine GO membrane. Interlayer distance of 1 :9 Gr- GO membrane
increases to 10 A, whereas it is 14 A for the pristine GO membrane
after soaking in water. Similarly, significant decrease (~ 100 to
1000 times) in the permeation rate for K<+>, Na<+>and
Li<+>ions is observed in the case of 1 :9 Gr-GO membranes.
The water permeation rate through GO and Gr-GO membrane has also
been measured by monitoring the osmotic height difference and it
was found that addition of graphene to GO membrane did not change
the osmotic height difference, indicating similar water permeation
rate for both GO and Gr-GO membranes.
[001 13] NaCI salt rejection properties of 1 :9 Gr-GO membranes
were further measured using forward osmosis technique by keeping
concentrated sugar solution as a draw solute. Salt rejection was
calculated using the equation 1-Cp/Cf where Cpis the concentration
of NaCI in transmitted water and Cf is the concentration of NaCI
in feed side. This analysis yields 96% salt rejection for the 1 :9
Gr-GO membranes. The salt rejection of GO only membranes is around
70%.
Graphene Oxide Membranes --
Select Patents
US2017036172
ENHANCED GRAPHENE OXIDE MEMBRANES AND METHODS FOR MAKING
SAME
US2017036166
METHODS RELATING TO ISOTOPIC WATER FILTRATION
US2017015483
GRAPHENE OXIDE NANOCOMPOSITE MEMBRANE HAVING IMPROVED GAS
BARRIER CHARACTERISTICS AND METHOD FOR MANUFACTURING THE SAME
US2017014778
GRAPHENE OXIDE NANOCOMPOSITE MEMBRANE FOR GAS SEPARATION,
REDUCED GRAPHENE OXIDE NANOCOMPOSITE MEMBRANE, AND METHOD FOR
MANUFACTURING THE SAME
US2016354729
MEMBRANES COMPRISING GRAPHENE
US2016297693
OSMOSIS
CN106000127
Carbon-containing seawater desalination film, preparation
method and application thereof
CN106032072
Method for preparing graphene oxide film and flexible
non-contact capacitive sensor
WO2016171490
GAS BARRIER MEMBRANE USING GRAPHENE OXIDE PREPARED BY SOLUTION
MIXING METHOD, AND METHOD FOR PREPARING SAME
CN105883781
Preparation method of large-area reduced graphene oxide
membrane
CN105883781
Preparation method of large-area reduced graphene oxide
membrane